Over the centuries, physicists have made giant strides in understanding and predicting the physical world by connecting phenomena that look very different on the surface.
One of the great success stories in physics is the unification of electricity and magnetism into the electromagnetic force in the 19th century. Experiments showed that electrical currents could deflect magnetic compass needles and that moving magnets could produce currents.
Then physicists linked another force, the weak force, with that electromagnetic force, forming a theory of electroweak interactions. Some physicists think the logical next step is merging all four fundamental forces—gravity, electromagnetism, the weak force and the strong force—into a single mathematical framework: a theory of everything.
Those four fundamental forces of nature are radically different in strength and behavior. And while reality has cooperated with the human habit of finding patterns so far, creating a theory of everything is perhaps the most difficult endeavor in physics.
“On some level we don't necessarily have to expect that [a theory of everything] exists,” says Cynthia Keeler, a string theorist at the Niels Bohr Institute in Denmark. “I have a little optimism about it because historically, we have been able to make various unifications. None of those had to be true.”
Despite the difficulty, the potential rewards of unification are great enough to keep physicists searching. Along the way, they’ve discovered new things they wouldn’t have learned had it not been for the quest to find a theory of everything.

United we hope to stand
No one has yet crafted a complete theory of everything.
It’s hard to unify all of the forces when you can’t even get all of them to work at the same scale. Gravity in particular tends to be a tricky force, and no one has come up with a way of describing the force at the smallest (quantum) level.
Physicists such as Albert Einstein thought seriously about whether gravity could be unified with the electromagnetic force. After all, general relativity had shown that electric and magnetic fields produce gravity and that gravity should also make electromagnetic waves, or light. But combining gravity and electromagnetism, a mission called unified field theory, turned out to be far more complicated than making the electromagnetic theory work. This was partly because there was (and is) no good theory of quantum gravity, but also because physicists needed to incorporate the strong and weak forces.
A different idea, quantum field theory, combines Einstein’s special theory of relativity with quantum mechanics to explain the behavior of particles, but it fails horribly for gravity. That’s largely because anything with energy (or mass, thanks to relativity) creates a gravitational attraction—including gravity itself. To oversimplify somewhat, the gravitational interaction between two particles has a certain amount of energy, which produces an additional gravitational interaction with its own energy, and so on, spiraling to higher energies with each extra piece.
“One of the first things you learn about quantum gravity is that quantum field theory probably isn’t the answer,” says Robert McNees, a physicist at Loyola University Chicago. “Quantum gravity is hard because we have to come up with something new.”
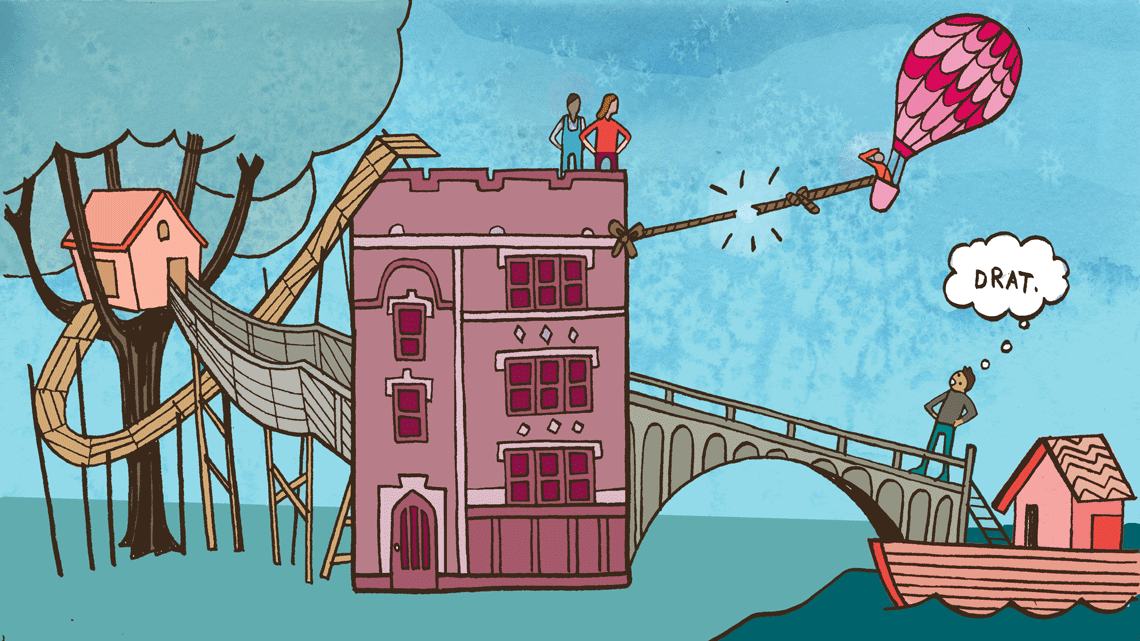
An evolution of theories
The best-known candidate for a theory of everything is string theory, in which the fundamental objects are not particles but strings that stretch out in one dimension.
Strings were proposed in the 1970s to try to explain the strong force. This first string theory proved to be unnecessary, but physicists realized it could be joined to the another theory called Kaluza-Klein theory as a possible explanation of quantum gravity.
String theory expresses quantum gravity in two dimensions rather than the four, bypassing all the problems of the quantum field theory approach but introducing other complications, namely an extra six dimensions that must be curled up on a scale too small to detect.
Unfortunately, string theory has yet to reproduce the well-tested predictions of the Standard Model.
Another well-known idea is the sci-fi-sounding “loop quantum gravity,” in which space-time on the smallest scales is made of tiny loops in a flexible mesh that produces gravity as we know it.
The idea that space-time is made up of smaller objects, just as matter is made of particles, is not unique to the theory. There are many others with equally Jabberwockian names: twistors, causal set theory, quantum graphity and so on. Granular space-time might even explain why our universe has four dimensions rather than some other number.
Loop quantum gravity’s trouble is that it can’t replicate gravity at large scales, such as the size of the solar system, as described by general relativity.
None of these theories has yet succeeded in producing a theory of everything, in part because it's so hard to test them.
“Quantum gravity is expected to kick in only at energies higher than anything that we can currently produce in a lab,” says Lisa Glaser, who works on causal set quantum gravity at the University of Nottingham. “The hope in many theories is now to predict cumulative effects,” such as unexpected black hole behavior during collisions like the ones detected recently by LIGO.
Today, many of the theories first proposed as theories of everything have moved beyond unifying the forces. For example, much of the current research in string theory is potentially important for understanding the hot soup of particles known as the quark-gluon plasma, along with the complex behavior of electrons in very cold materials like superconductors—something seemingly as far removed from quantum gravity as could be.
“On a day-to-day basis, I may not be doing a calculation that has anything directly to do with string theory,” Keeler says. “But it’s all about these ideas that came from string theory.”
Finding a theory of everything is unlikely to change the way most of us go about our business, even if our business is science. That’s the normal way of things: Chemists and electricians don't need to use quantum electrodynamics, even though that theory underlies their work. But finding such a theory could change the way we think of the universe on a fundamental level.
Even a successful theory of everything is unlikely to be a final theory. If we’ve learned anything from 150 years of unification, it’s that each step toward bringing theories together uncovers something new to learn.